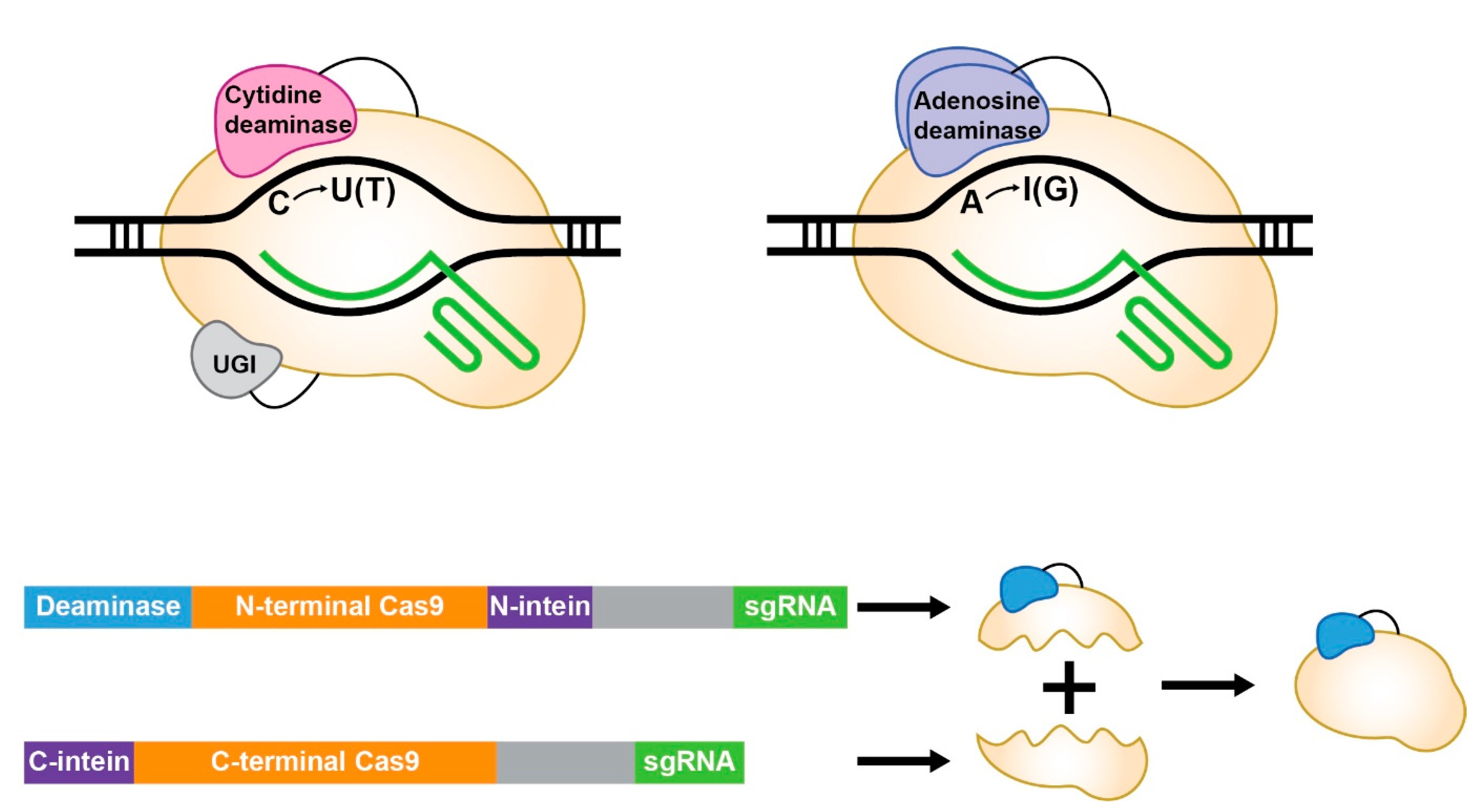
Base editors—tools that precisely alter single DNA nucleotides without breaking both strands—offer a safer path for gene therapy than traditional CRISPR-Cas systems. Their ability to correct point mutations without double-strand breaks reduces risks like unintended genomic changes, making them valuable for treating diseases tied to single-base errors. Yet, their size poses a significant challenge. Standard base editors, built on Cas9 and deaminases, often exceed the capacity of adeno-associated virus (AAV) vectors. For labs working on developing gene therapies, this size mismatch can complicate workflows and limit clinical potential. This post, inspired by a recent review in the International Journal of Molecular Sciences by Beomjong Song (2025), explores efforts to make base editors smaller. It’s a practical look at the problem and the solutions, because if you’ve struggled with AAV payloads, you’re not alone.
Why Base Editor Size Is a Hurdle: The AAV Bottleneck
Base editors combine a Cas protein (e.g., SpCas9) with a deaminase (e.g., APOBEC1 for cytosine edits, TadA for adenine) to target and modify DNA bases, guided by RNA to specific sites. This assembly, while precise, is large: a typical SpCas9-based editor exceeds 5.2 kilobases (kb), while AAVs cap out at ~4.7 kb. Exceeding this limit means splitting the editor across two AAVs, which doubles the viral load, reduces transduction efficiency, and increases manufacturing complexity. Even partial fits push out other elements, like promoters, shrinking design flexibility. For gene therapy, where every base pair counts, this bottleneck slows progress from lab to clinic. Song’s review highlights how researchers are addressing this by downsizing base editors to fit AAVs without losing their editing power.
Strategies to Shrink Base Editors: Making Precision Fit
Efforts to reduce base editor size focus on four main approaches, each with strengths and trade-offs. Here’s a detailed look at how these strategies work, grounded in recent research.
Smaller Cas Proteins: Trading Size for Scope
One approach replaces the standard SpCas9 (1368 amino acids, ~4.1 kb) with smaller Cas orthologs. Options like SaCas9 (1053 amino acids, ~3.2 kb), NmeCas9 (1082 amino acids, ~3.2 kb), or St1Cas9 (1121 amino acids, ~3.4 kb) reduce the DNA footprint by 700-900 base pairs. This size decrease fits base editors within AAV limits when paired with a deaminase. However, these proteins recognize stricter PAM sequences. For example, SaCas9 needs NNGRRT (vs. SpCas9’s NGG), NmeCas9 needs NNNNCC, and St1Cas9 needs NNAGAAW, limiting their targetable sites. Studies show SaCas9-based editors maintain high editing efficiency in mammalian cells, though off-target effects may rise due to less flexible targeting. For labs, this swap simplifies delivery but requires sequence compatibility checks upfront.
Trimming Deaminases: Reducing Bulk, Retaining Function
Another method trims non-essential regions of deaminases. Cytosine base editors (CBEs) use rat APOBEC1 (0.7 kb), while adenine base editors (ABEs) rely on evolved TadA (0.5 kb). Both can be shortened. For example, removing linker domains or non-catalytic segments in TadA creates “mini ABEs,” saving ~200-300 base pairs with minimal impact on editing rates. Research cited by Song demonstrates these truncated versions retain specificity in cell lines, though some efficiency dips occur (e.g., 10-20% lower conversion). CBEs with shortened APOBEC1 show similar results: functional, but needing optimization. This approach keeps the editor intact in one AAV, simplifying production, though labs must validate edits for their specific targets.
Splitting Base Editors: Two AAVs, One Goal
When downsizing isn’t enough, splitting base editors across two AAVs offers a workaround. The Cas protein and deaminase are divided into N- and C-terminal halves, each fused to intein sequences that reassemble inside cells. This method fits each half (~2.5-3 kb) into an AAV, alongside guide RNA and regulatory elements. Studies show split CBEs and ABEs correct mutations like DMD exon skips in cell models, but efficiency drops to ~50% of full editors due to incomplete reassembly or uneven transduction. Manufacturing doubles and dosing increases, challenging clinical scale-up. Still, it’s a viable bridge until smaller designs mature, offering labs a current option for oversized editors.
Beyond AAV: Exploring New Delivery Methods
Alternative delivery systems sidestep AAV limits entirely. Lentiviral vectors handle larger cargos (~9 kb), delivering full base editors without splits that are useful for ex vivo edits like CAR-T cells, but their integration risks limit in vivo use. Nanoparticles, such as lipid or gold-based carriers, encapsulate base editor mRNA or proteins, bypassing size constraints. Early trials have been successful in correcting liver mutations in mice, though delivery consistency lags behind AAV’s tissue specificity. Hydrodynamic injections target organs like the liver but aren’t scalable for humans. These methods expand possibilities, yet AAV remains the clinical standard and labs still weigh safety and efficiency when exploring beyond it.
What It Means for Gene Therapy: From Lab to Clinic
Shrinking base editors unlocks real-world impact. Smaller SaCas9-based editors have been able to correct sickle cell mutations in mouse hematopoietic cells, restoring hemoglobin function with one AAV. Split ABEs have been shown to restore dystrophin in DMD cell lines, hinting at muscle therapy potential, though achieving practical efficiency still needs work. These advances target rare diseases with single-nucleotide fixes, where precision trumps broad cuts. Fitting more editors into AAVs can cut costs and complexity, which is key for therapies reaching patients. This gives research teams significant flexibility in their strategy: one vector, one dose, closer to clinical reality. The challenge remains balancing size reduction with editing accuracy and progress here could redefine gene therapy’s reach.
Looking Ahead: Smarter, Smaller, Sooner
Base editor downsizing is gaining momentum. Combining smaller Cas proteins with trimmed deaminases could yield sub-4.7 kb editors, ready for AAV and clinical trials. AI tools might soon predict optimal designs, scanning sequences and studies for precision cuts, streamlining the process further. Song’s review shows a field on the cusp: as these strategies mature, gene therapy becomes more accessible, not just for well-funded teams but for any lab with a vision.
Source: Song, B. Efforts to Downsize Base Editors for Clinical Applications. Int. J. Mol. Sci. 2025, 26(5), 2357. Available open-access at https://doi.org/10.3390/ijms26052357.
Need vectors sized right? Reach out: customer@ambryon.com.